Introduction
Implantable cardioverter-defibrillators (ICD) demonstrate a mortality reduction in patients at risk for sudden cardiac death.1–4 Transvenous lead placement with a subcutaneous, pectoral pulse generator has been the standard approach for ICD implantation for the past two decades,5 and have a high rate of successful implantation and a very low risk of in-hospital mortality.6 Despite increased operator experience, improvements in technology and surgical technique, there are risks inherent in the surgical procedure and transvenous ICDs. Recent data from the US National Cardiovascular Data Registry (NCDR) demonstrate an adverse event rate of 2.2 % and a 1.56 % major adverse event rate (defined as death, cardiac arrest, lead dislodgment, hemothorax, pneumothorax, tamponade, urgent cardiac surgery, myocardial infarction, cerebral vascular accident, and set screw problem).7
Although there is a low overall risk of periprocedural complications, additional long-term risks are associated with transvenous ICDs. Rates of infection are reported near 1.5 %,7,8 and may include cardiac and non-cardiac sites. Lead complication rates approach 10 % in randomized controlled trials8 and the annual failure rate increases proportionally with time after implantation.9 Reasons for failure include dislodgment, insulation defects, fracture, loss of capture, inability to sense appropriately, or abnormal impendence. In an effort to address these concerns, an entirely subcutaneous ICD (Cameron Health/Boston Scientific) was developed gaining Conformité Européene (CE) approval in Europe in 2008 and Food and Drug Administration (FDA) approval in 2012.5
Subcutaneous Implantable Cardioverter-defibrillator System, Screening, and Implantation
The subcutaneous ICD system consists of a pulse generator that is connected to a lead containing a single high-voltage, low-impedance shock coil and two sensing electrodes. The device senses from one of three different vectors: proximal ring to generator (primary); distal tip electrode to generator (secondary); and distal tip to proximal ring electrode (alternate). The volume of the first generation of the device is 69 ml, with a mass of 145 g.10 The second generation is slightly smaller, with a volume of 59.5 mL and mass of 130 g.11
Preliminary short-term trials beginning in 2001 sought to identify the most effective electrode position for the subcutaneous ICD (S-ICD) on the basis of anatomical landmarks. Four different electrode positions were tested and the most effective location was a left lateral pulse generator with an 8-cm coil electrode positioned to the left of the sternum.12 Patients under consideration for S-ICD implantation should undergo a preimplant ECG to assess for QRS-T wave morphology to reduce double counting of T-waves resulting in inappropriate defibrillations.13 ECG screening is necessary to ensure patient compatibility with one of the three vectors utilized with the S-ICD device. In the largest registry to date, patients were required to pass the screening in at least one vector in the supine and standing position. Of the 1637 patients evaluated, full data on all three vectors were available for review in 1622 patients. ECG vector screening was acceptable in two and all three vectors in 93.8 % and 51.4 % of patients, respectively. Lower BMI or higher left ventricular ejection fraction (LVEF) were predictive characteristics of patients only passing one vector.14
The generator is implanted between the mid-axillary and anterior axillary lines connected to the electrode, which is tunneled typically 1 to 2 cm to the left of and parallel to the sternum.15 Figure 1 illustrates the anatomic location as well as the sensing vectors of the S-ICD system. In early implantations, the lead was tunneled via an inferior and superior parasternal incision (three-incision technique). In a recent trial, however, the majority of implantations were via the two-incision technique, requiring only an inferior sternal incision.14 A study of 69 patients implanted with an S-ICD at three German centers demonstrated a mean implantation time of 70.8 ± 27.9 min, which did not differ significantly from conventional ICD implantation times.16 Sedation strategies have varied widely across trials, with the rates of general anesthesia use ranging from 47 %17 to 100 %.18 In the recently published US S-ICD post-market approval study (S-ICD PAS), general anesthesia was utilized in 64.1 % of implantations.14 Arrhythmia termination is typically tested using 65 J shocks at the conclusion of the procedure. Once implanted, the device output is a non-programmable 80 J shock. The device automatically reverses the polarity of the shock if the initial attempt is unsuccessful. Maximum therapy consists of five defibrillations.18 Aside from 30 sec of post-shock asystole demand pacing, the device has no anti-bradycardic or anti-tachycardia (ATP) functions.15
The 2017 American Heart Association/American College of Cardiology/Heart Rhythm Society guidelines for management of patients with ventricular arrhythmias and the prevention of sudden cardiac death recommend S-ICD implantation in patients meeting criteria for ICD whom:
- have inadequate vascular access or an unacceptable risk of infection (Class I, level of evidence [LOE] B- non-randomized [NR]); or
- pacing for bradycardia, termination of ventricular tachycardia, or CRT is neither needed nor anticipated (Class IIa, LOE B-NR).
S-ICD implantation is not recommended in patients in whom pacing for bradycardia, ATP, or CRT is necessary or envisioned (Class III, LOE B-NR).13
Populations Studied
Evaluation of the clinical trials investigating the S-ICD system requires knowledge of the population analyzed. Early studies commonly included a high proportion of niche populations who were younger with little or no structural heart disease and fewer co-morbidities than most series of patients receiving transvenous ICDs. Mean age ranged from 42 to 53 years.16–22 Two publications reported median ages of 20 and 33 years.23,24 The majority of early cohorts consisted of fewer than 120 patients.16–19,23,24 Subsequently, the results from the EFFORTLESS (Evaluation oF FactORs ImpacTing Clinical Outcome and Cost EffectiveneSS of the S-ICD) registry were reported on a population of 985 S-ICD recipients.25 Within these studies, the prevalence of primary electrical heart disease ranged from 20 %16 to 75 %.23 When reported, mean LVEF was greater than 35 % in all cases16–22,25 and greater than 40 % in five studies.16–18,21,25 Primary prevention was the initial indication for implantation in 42 %18 to 79 %20 of cases. Men represented at least 70 % of each cohort16–22,25 in all but one trial, where men accounted for 9 out of 16 patients.23 Although these studies provided valuable information regarding the S-ICD system, as noted above the cohorts studied are not entirely representative of typical ICD patients. Accordingly, these differences should be considered when extrapolating the results to broader populations.
Two recent publications have analyzed S-ICD implantation in larger populations with higher prevalence of concomitant co-morbidities. Friedman et al retrospectively analyzed NCDR ICD data from 2012 to 2015 and performed a propensity matched analysis of 5760 patients in a 1:1:1 fashion to compare outcomes among patients implanted with S-ICD, single-chamber, and dual chamber ICD. Patients implanted with S-ICD were found to be more often younger, female, African American, and dialysis dependent, and were more likely to have experienced prior cardiac arrest when compared with more traditional ICD counterparts. Mean LVEF was 32 % and the prevalence of dialysis dependence was 20 % in the S-ICD cohort.26 A second study, mandated following FDA approval (PAS study), prospectively enrolled and followed patients who received an S-ICD. This population consisted of 1637 S-ICD recipients, 13.4 % of whom were on dialysis. Mean LVEF (32 %) was also lower than other prior S-ICD studies and patients within this study had more co-morbidities than prior publications. The majority of patients had both heart failure and hypertension and over one-third had diabetes. Patients with an LVEF < 35 % and heart disease constituted approximately 75 % of all patients. Additionally, a lower number of patients with inherited channelopathies were enrolled.14 The PAS study demonstrated that in contemporary clinical practice, the S-ICD population has shifted more from selected niche population to typical ICD cohorts. Table 1 compares the populations studied from the S-ICD Clinical Investigation (IDE), EFFORTLESS, and S-ICD PAS trials.
Safety
As with any medical procedure, there are risks inherent in the implantation of ICDs. However, these risks differ among the types of ICDs implanted. Complications associated with the implantation of transvenous ICDs include pneumothorax, hemothorax, nerve or vascular damage, hematoma, infection, lead dislodgment or malfunction, cardiac perforation, and tamponade. A meta-analysis of traditional ICD randomized controlled trials demonstrated a complication rate of 9.1 %, though a few studies included devices implanted via a thoracotomy. This was compared with a ‘real-world’ complication rate of 3.08 % for ICD implantation derived from NCDR between 2006 to 2010.8 Discrepancy between the two rates may be, at least somewhat, accounted for by the intrinsic nature of a comparison between randomized controlled trials and registry data. It is likely that the NCDR registry underestimates long-term complications given the nature of the data collection post implant. A second publication also corroborated the reported complication rate from the NCDR data during that same time period.27 The rates of the most common adverse events were as follows: lead dislodgement 1.02 %, hematoma 0.86 %, pneumothorax 0.44 %, and cardiac arrest 0.29 %.27 Other studies quote slightly higher rates of pneumothorax and hematoma.6,8 NCDR data from 2010 to 2011 demonstrate an even lower adverse event rate.7
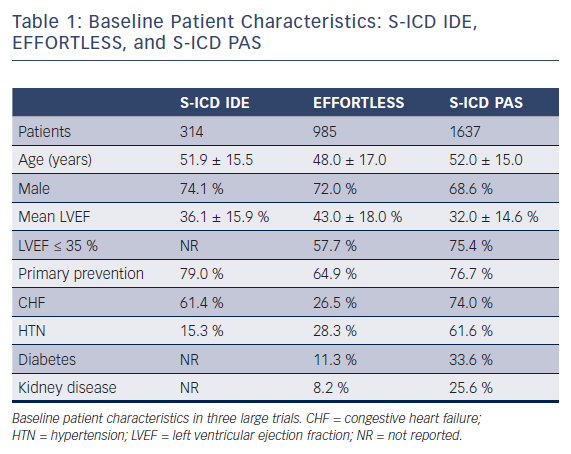
Although ICD periprocedure adverse event rates are acceptably low, the complications associated with a chronic indwelling transvenous ICD, if present, often lead to significant comorbidity and reoperation. Infection has been reported in 1.5 % of transvenous devices.7,8 The infection may range from localized pocket or wound infection to fulminant endocarditis. Most patients ultimately require explantation in order to treat the infection successfully. Lead malfunction or defects typically require reoperation with implantation of additional transvenous leads and abandonment of the problematic lead. Annual failure rates of transvenous leads increase with time after implantation. The estimated lead survival rate at 5 and 8 years is 85 % and 60 %, respectively. Annual failure rate of leads at least 10 years old is 20 %.9 The S-ICD system was designed, in part, as a way to circumvent many of the complications associated with transvenous ICD implantation.
Safety analyses of the S-ICD and comparison with that of conventional ICDs are crucial to its development and clinical acceptance. Earlier S-ICD trials demonstrated a higher rate of complications than later ones, reflective of increased operator experience and improved technology. In the initial trial describing the S-ICD system, thirteen of 55 patients had device related adverse events.12 In a Dutch cohort of 118 patients, sixteen experienced complications and adverse events were more frequent in the initial 15 implantations.17 In the S-ICD IDE study, which had rigorous FDA oversight, Weiss et al reported a 180-day complication-free rate of 92.1 % for all complications and, more specifically, 99 % for complications caused by the S-ICD system.20 Early results from EFFORTLESS reported similarly low complications with complication-free rates of 97 % and 94 % at 30 and 260 days, respectively.21 Recently, a large registry corroborated high complication-free rates of the S-ICD.14 A propensity-matched analysis found that the in-hospital complication rates associated with S-ICD (0.9 %) were not significantly different than that of single chamber or dual chamber ICDs.26 A second analysis found that complication rates between S-ICD and transvenous ICDs were similar, but that the nature of the complications was different. S-ICDs reduced lead complication rates but it was at the cost of non-lead related complications.28 Reported rates of infection and hematoma formation in S-ICDs14,22 are similar to rates previously reported in conventional ICDs.7,8,27 However, importantly, S-ICD infections are localized and have not been associated with bacteremia or systemic involvement. Improvement in the complication rate suggests a learning curve associated with the implantation of S-ICDs. A pooled cohort from the IDE study and EFFORTLESS registry demonstrates a significantly decreased complication rate with more experienced operators.29 Technology advances have also contributed to decreased S-ICD adverse events. Parasternal lead migration was encountered frequently in early clinical trials; however, in at least two trials, no further lead migration was observed following the introduction of a xiphoid suture sleeve to the operative protocol.17,19
Inappropriate shocks are associated with worsened quality of life, increased healthcare costs,30,31 so minimizing such events has been an area of intense study for ICDs. The cause of inappropriate shocks with S-ICD have been inappropriate sensing of myopotentials, T-wave oversensing, changes in QRS morphology, or failure to discriminate supraventricular tachycardia (SVT). In one study, no further inappropriate shocks were observed following a software update specifically addressing myopotential oversensing.19 Other software updates addressing T-wave oversensing, changes in sensing vectors during exercise, or the addition of new templates have led to reductions in inappropriate therapy.17
For transvenous ICDs, conservative programming of tachycardia treatment zones by prolonging detection duration or increasing threshold rates for therapy has been shown to not only reduce inappropriate shocks, but also improve mortality.32 The Subcutaneous versus Transvenous Arrhythmia Recognition Testing (START) study demonstrated that the S-ICD discriminated SVT more effectively than transvenous ICD systems.33 Thus, the use of dual zone programming employing a conditional zone (rate plus discriminators) markedly reduces inappropriate shocks.34 Continued reductions in inappropriate shocks as well as improvements in device implantation and technique with new generations of the S-ICD device will likely lead to an even more acceptable safety profile. These differences in the types of lead complications and inappropriate shocks between transvenous and subcutaneous ICDs has been further supported by a recent meta-analysis.35
The safety profiles of a device are also affected by longevity. A device with a shorter battery life or time to the elective replacement interval (ERI) exposes the patient to more procedures with their intrinsic risks. Conventional ICDs implanted after 2002 were found to have a mean battery life of 5.6 years.36 Although device longevity varies somewhat with manufacturer and programmed mode, overall longevity of devices continues to improve with more recently implanted devices.37 The manufacturer of the S-ICD initially projected device longevity of 5 years.10 A nearly 6-year follow-up of 55 patients enrolled in the European Regulatory Trial demonstrated a device replacement rate of 47 %. The majority of devices were replaced on ERI (81 %) and the median time for device replacement was 5 years. Premature battery depletion occurred in 9 % of the initial S-ICD cohort leading to a field safety notification regarding a battery manufacturing issue. Following correction, premature battery depletion was observed in 0.6 % in the IDE trial and 0.2 % in the EFFORTLESS registry.38 Published rates of premature battery depletion in transvenous ICDs are 8–9 %.39,40 The second generation S-ICD system has manufacturer projected longevity of over 7 years,11 though this will need to be validated with subsequent analyses.
Efficacy
S-ICD devices are effective in appropriately sensing and terminating VT/VF. Conversion testing is typically performed immediately following implantation with induction of VT/VF and a 65 J shock providing an adequate (15 J) safety margin.12,16,18,19 An early trial comparing temporary S-ICD systems with transvenous ICDs found that conversion efficacy was similar, though S-ICD systems had higher defibrillation thresholds.12 Moreover, the START study demonstrated no significant differences in ventricular arrhythmia detection for S-ICDs and transvenous devices.33 A larger study of 899 episodes of induced VT/VF established a 99.8 % rate of successful VT/VF detection and defibrillation. In those instances where VT/VF was successfully detected, successful defibrillation was obtained in 100 % of patients.20 An early study of 40 consecutive S-ICD patients did demonstrate a low conversion efficacy with the initial shock; however, 96.4 % had successful conversion within the five allotted shocks.18 Recent large trials further corroborate the ability of the S-ICD system to successfully defibrillate induced VT/VF.14,26 Failure of conversion with the first shock is predicted by patient height and BMI.14 Conversion testing has also been performed ≥ 150 days after implantation. Of the 75 patients with evaluable results, 72 (96 %) were successfully converted at 65 J. The three other patients were successfully converted at 80 J.20 Mean time to therapy has been reported at 14.6 and 19.2 sec20,22 and is in line with the current paradigm for programming of defibrillation therapy for transvenous systems.30
Induction of VT/VF and conversion testing indicates proper device functioning in a controlled setting. However, demonstration of termination of spontaneous episodes is obligatory to show the true benefit of ICDs. Weiss et al reported 119 spontaneous VT/VF episodes in 21 patients. There were 38 discrete VT/VF episodes and 81 that occurred during VT/VF storms. The first shock conversion rate for the discrete episodes was 92.1 % and all but one was terminated with ≥ 1 shocks. The exception was an episode of monomorphic VT, which terminated spontaneously while the device was charging for a second shock.20 In the EFFORTLESS registry, the overall successful conversion rate for spontaneous episodes was 97.4 %.25 Other investigations have found similarly high rates of successful first shock17 and overall shock efficacy.22
Limitations
Despite evidence establishing the safety and efficacy of the S-ICD, the device does have limitations. The system does not have the ability to provide chronic anti-bradycardic, anti-tachycardic pacing, or CRT. It is able to provide up to 30 sec of post-shock asystole pacing at a rate of 50 bpm.15 The inability to provide pacing chronically emphasizes the importance of appropriate patient screening to exclude those patients with, or who may develop, bradycardic indications. In the 3-year follow-up of the EFFORTLESS registry, the S-ICD was explanted for the indication of bradycardia in 0.1 %, ATP in 0.5 %, and CRT in 0.4 % of patients.25 Low rates of S-ICD explantation and transition to transvenous devices for bradycardia, CRT, or ATP likely reflect the importance of proper patient selection.
Conclusion
The S-ICD device is a safe and effective alternative to contemporary transvenous ICDs in selected patients. Additionally, new studies have demonstrated both safety and efficacy in broader, sicker populations.14,26 This is being studied in even more detail in the UNTOUCHED trial of primary prevention patients with a reduced ejection fraction.41 Though direct randomized comparisons between the two systems are currently unavailable, the Prospective, Randomized Comparison of Subcutaneous and Transvenous Implantable Cardioverter-Defibrillator Therapy (PRAETORIAN) trial is ongoing.42 In selected patients, and arguably most, who qualify for ICD therapy without an indication for pacing, CRT, or ATP, the subcutaneous ICD system should be considered.