Survival rates among patients diagnosed with cancer have improved dramatically over the past few decades. In 2016, there were an estimated 16 million cancer survivors in the United States, a number expected to increase to 20 million over the subsequent decade.1 One-third of these patients will survive at least 5 years after their initial cancer diagnosis, largely due to cancer therapies that are more efficacious and better tolerated. However, many of these treatments have the potential to contribute to cardiac toxicities that can affect quality of life, and in many cases, overall survival (see Table 1). Moreover, the development of cardiac toxicities can prevent clinicians from achieving effective doses of chemotherapy or prompt them to choose suboptimal cancer treatment regimens. At present, data to guide management of chemotherapyinduced cardiac toxicities stem from observational studies and small randomized trials, and the majority of recommendations are driven by clinical expertise (see Table 2). Here we will review a number of antitumor agents that have been associated with significant cardiac toxicity, from traditional cytotoxic chemotherapies that have been in use for decades to the newer targeted therapies and immune checkpoint inhibitors.
Cytotoxic Chemotherapy
Anthracyclines
The anthracyclines were the first major class of chemotherapies to be associated with cardiac toxicity. Anthracyclines are derivatives of Streptomyces that were originally discovered to have antitumor properties in the 1960s2 and first linked to cardiac toxicity in the early 1970s.3 Given the extensive data supporting their efficacy, anthracyclines are commonly used for the treatment of breast cancer, leukemia, lymphoma, and sarcoma, despite the advent of newer targeted therapies. The anthracycline doxorubicin (Adriamycin®) is well recognized for increasing the risk of cardiomyopathy, with the risk of congestive heart failure (CHF) increasing in proportion to the lifetime cumulative dose administered.4 Although the highest rates of cardiac toxicity have been described with cumulative doses exceeding 400 mg/m2, CHF has been reported in patients exposed to much lower doses.5 A recent study suggested that 9 % of patients treated with anthracyclines experienced cardiotoxicity, defined as a decline in left ventricular ejection fraction by >10 percentage points to an absolute value of <50 % within the first year following completion of anthracycline-based chemotherapy.6 Importantly, the risk of anthracycline-associated cardiotoxicity increases substantially when combined with other common cancer treatments such as chest radiation and trastuzumab.7
Despite decades of investigation, the precise mechanisms of anthracycline-induced cardiotoxicity have not been well defined. Prior work has focused primarily on the role of oxidative stress.8 Doxorubicin forms a semiquinone radical that reacts rapidly with oxygen to form a superoxide anion, leading to the production of hydroxyl radicals in the presence of heavy metals such as iron.9 In addition, doxorubicin binds directly to iron to generate free radicals.10 Doxorubicin-induced oxidative stress has been proposed to be mediated by mitochondrial iron accumulation,11 doxorubicin’s interaction with the beta isoform of topoisomerase II (Top2beta),12 and autophagy.13 However, despite evidence implicating oxidative stress as a common downstream feature of doxorubicin-induced cardiomyopathy, antioxidants are ineffective in preventing cardiotoxicity in patients.14,15 Similarly, iron chelators such as deferasirox have failed to protect against doxorubicin cardiotoxicity in preclinical models.16 Liposomal formulations can be used as alternatives to traditional anthracyclines with reduced rates of cardiotoxicity.17 These agents preferentially enter into the leaky microvasculature of tumors, but have limited extravasation into cardiomyocytes.18 Given the increased cost of liposomal formulations, as well as the increased risk of mucositis and hand–foot syndrome, they are used primarily in the setting of refractory or metastatic disease where high doses of anthracyclines are required.
Based on extensive literature supporting the use of neurohormonal blockade in other types of cardiomyopathy, a few small, randomized clinical trials have been performed to examine the role of beta blockade and angiotensin-receptor blockade for the primary prevention of anthracycline-induced LV dysfunction.19–23 Currently, dexrazoxane is the only U.S. Food and Drug Administration (FDA)-approved drug used clinically to prevent doxorubicin-induced cardiomyopathy. It is believed to chelate intracellular iron, block iron-assisted oxidative radical production, and inhibit Top2beta.11,12,24,25 In practice, however, the use of dexrazoxane has been limited by concerns that it may interfere with doxorubicin’s ability to kill tumor cells26 and may also induce secondary malignancies.27 As such, the FDA has restricted its use to patients with metastatic breast cancer who have received a cumulative lifetime dose of at least 300 mg/m2 of doxorubicin or an equivalent dose of other anthracyclines. However, subsequent trials have not suggested any decrease in antitumor efficacy with the use of dexrazoxane, and similar oncologic response rates were reported in a large Cochrane meta-analysis.28 Similarly, two subsequent reports of childhood survivors of acute lymphoblastic leukemia who were treated with dexrazoxane did not suggest any increase in the rate of secondary malignancies.29,30 Thus, many clinicians continue to prescribe dexrazoxane in patients receiving a high cumulative dose of anthracyclines, particularly in those with pre-existing cardiovascular disease or other risk factors for the development of cardiotoxicity.
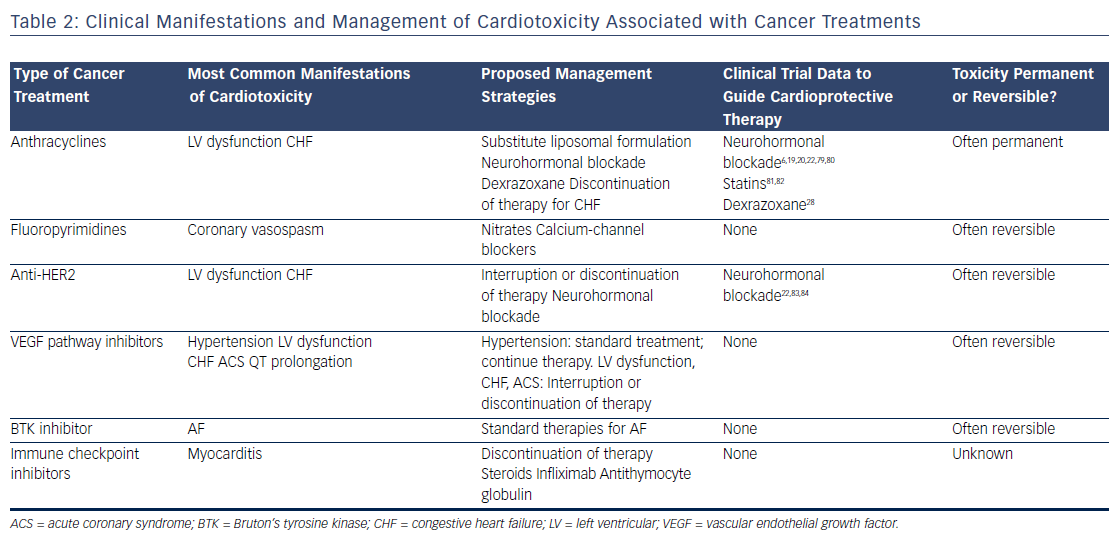
Fluoropyrimidines
The fluoropyrimidines 5-fluorouracil and its oral prodrug capecitabine are commonly used in the treatment of gastrointestinal malignancies. Coronary vasospasm leading to myocardial ischemia has been widely described in association with fluoropyrimidine treatment, typically manifested by the development of angina.31 Notably, the presence of pre-existing ischemic heart disease may increase the risk of developing fluoropyrimidine-induced cardiotoxicity.32 CHF, myocarditis, and ventricular arrhythmias have also been reported, suggesting a direct myocardial toxicity.33–35 Although cardiotoxicity is typically reversible on withdrawal of the offending agent, sudden death has been reported in the setting of fluoropyrimidine use.36 Proposed mechanisms of coronary vasospasm include protein kinase C-mediated vasoconstriction,37 impaired handling of reactive oxygen species,38 and direct endothelial toxicity.39 Calcium-channel blockers and nitrates are often prescribed for prophylaxis in patients suspected to have fluoropyrimidine-induced coronary vasospasm, although their efficacy in preventing subsequent episodes of vasospasm is debated.
Targeted Therapies
Anti-Human Epidermal Growth Factor Receptor 2 Therapy
Recent developments in molecular profiling have led to a rapid increase in the number of cancer therapies targeting specific tyrosine kinases that are overexpressed in tumor tissue, particularly those involved in growth factor signaling. At present, the most commonly used targeted therapies are those that inhibit the human epidermal growth factor receptor 2 (HER2), which is overexpressed in approximately 25 % of all breast tumors,40 as well as some gastrointestinal and other tumors. Currently available therapies targeting the HER2 receptor include two humanized monoclonal antibodies, trastuzumab and pertuzumab, as well as the small-molecule inhibitor lapatinib. Cardiotoxicity arising from these therapies is most likely an on-target effect, as signaling through the HER2/neuregulin pathway is important in the cardiomyocyte response to injury during myocardial ischemia41 and anthracycline exposure.42
Initial studies demonstrated rates of LV dysfunction as high as 8 % for trastuzumab alone and 30 % for concomitant trastuzumab and anthracycline therapy,43 although estimates of cardiac toxicity have varied widely in subsequent clinical trials and community-based observational studies. Recent data suggest that combination therapy incorporating both trastuzumab and pertuzumab does not seem to increase the risk of cardiotoxicity.44 Of the three agents currently in use, lapatinib appears to be associated with the lowest risk of cardiotoxicity.45 Interruption or cessation of anti-HER2 therapy often results in recovery of LV function, although breast cancer outcomes may be affected as a result.46 If trastuzumab is interrupted due to cardiotoxicity, many patients experience recovery of LV function and can be safely re-challenged to complete a course of therapy. Late cardiotoxicity does not seem to occur after cessation of anti-HER2 therapy, unless patients are re-exposed to cardiotoxic chemotherapy.47
Vascular Endothelial Growth Factor Pathway Inhibitors
Inhibitors of the vascular endothelial growth factor signaling pathway are becoming increasingly used in the treatment of a number of malignancies, in particular renal cell carcinoma and gastrointestinal stromal tumor. Currently available agents range from monoclonal antibodies such as bevacizumab to small-molecule inhibitors such as sunitinib and sorafenib. Most of the small-molecule inhibitors in use today are multitargeted and thus affect the activity of a number of different tyrosine kinases, making it difficult to ascertain whether cardiotoxicity is related to on-target and/or off-target effects. In general, agents with a broad kinome-binding profile such as sunitinib have been associated with high rates of hypertension and LV dysfunction, although these effects are often reversible on discontinuation of the offending agent.48 It remains unclear whether the cardiomyopathy observed with these agents stems from direct toxicity to cardiomyocytes, or whether it is secondary to underlying vascular dysfunction and endothelial toxicity.49 Hypertension does appear to be an on-target effect, and in renal cell carcinoma, the development of hypertension correlates with increased efficacy of treatment and improved cancer outcomes.50 QT prolongation has also been observed with many of these agents51 and may be exacerbated by electrolyte abnormalities and other QT-prolonging medications such as antiemetics. In general, a prolongation of the corrected QT interval to >500 ms warrants a discussion of the risks and benefits of continuing therapy, although the underlying mechanisms and risk of developing torsades de pointes in this setting remain unclear.
Ibrutinib
The irreversible Bruton’s tyrosine kinase (BTK) inhibitor ibrutinib has been associated with high rates of AF. Ibrutinib is currently used for the treatment of relapsed or refractory chronic lymphocytic leukemia and mantle cell lymphoma as well as Waldenström macroglobulinemia. Initial clinical trials demonstrated rates of AF ranging 3–12 % in patients treated with ibrutinib.52,53 Notably, patients in these studies were older and had prior exposure to anthracyclines, factors that may have contributed to the high rates of AF observed. Preliminary work suggests that on-target effects may contribute to ibrutinib-mediated AF.54 However, newer BTK inhibitors have not been associated with increased rates of AF,55 suggesting an off-target mechanism. Although discontinuation of ibrutinib therapy is necessary in some patients, others respond well to standard treatments for AF and can continue ibrutinib at a full or reduced dose.56
Immune Checkpoint Inhibitors
The use of immune checkpoint blockade has become widespread for the treatment of metastatic melanoma57–59 and increasingly for other indications such as non-small cell lung cancer60 and renal cell carcinoma.61 Currently available agents include pembrolizumab, nivolumab, and atezolizumab, which target the programmed cell death protein-1 (PD-1) pathway, and ipilimumab, which targets cytotoxic T-lymphocyteassociated protein 4 (CTLA-4). These monoclonal antibodies modulate the T-cell-inhibitory responses that allow for tumor evasion from host immunity. As a result, treatment with these agents results in T-cell activation and proliferation, prompting a robust immune response against cancer cells.62
The cardiotoxicity of immune checkpoint inhibitors, while rare, has become increasingly important with the application of these therapies to expanding populations, especially when anti-PD-1 and anti-CTLA-4 agents are used in combination. Myocarditis is the predominant manifestation of cardiotoxicity, as described in initial clinical trials63,64 as well as case reports.65 A recent report highlighted two patients treated with combination immune checkpoint blockade and diagnosed with myositis as well as fulminant myocarditis; both patients died from cardiotoxicity.66 In these patients, endomyocardial biopsy demonstrated clonal T-cell infiltrates that were similar to those seen in tumor tissue. Preclinical models have suggested that modulation of the PD-1 pathway can lead to immune-mediated cardiovascular toxicity, primarily in the form of autoimmune myocarditis. Knockout of the PD-1 receptor in mice causes severe dilated cardiomyopathy characterized by high levels of immunoglobulin G autoantibodies that react specifically to cardiac troponin I.67,68 In mouse models of lupus and other experimentally induced inflammatory states, the PD-1 pathway has been recognized as an essential mediator of autoimmune myocarditis69–71 and has been similarly associated with high-titer autoantibodies against cardiac myosin.71 Proposed therapies include high-dose steroids, anti-tumor necrosis factor-alpha antibodies such as infliximab, and antithymocyte globulin, although there is currently no evidence to support an improvement in clinical outcomes with these measures.
Conclusion
Over the past decade, efficient, target-based development of antitumor agents has facilitated the introduction of several new cancer therapies, many of which are being approved for new types of malignancy each year. In 2016 alone, the FDA approved the use of targeted therapies and immune checkpoint inhibitors for 19 new indications.72 Many targeted cancer therapies are relatively well tolerated compared with conventional cytotoxic chemotherapy, enabling long-term use in some patients. Cardiologists will thus be faced with the challenge of managing a range of cardiac toxicities that can significantly impact patient morbidity and mortality. Efforts to understand the underlying mechanisms and molecular predictors of cardiotoxicity will be essential to identify patients at high risk and to guide decisions regarding cardioprotection. Collaboration between cardiologists and oncologists in managing cardiotoxicity will be of the utmost importance to ensure the best outcomes in patients receiving these therapies.